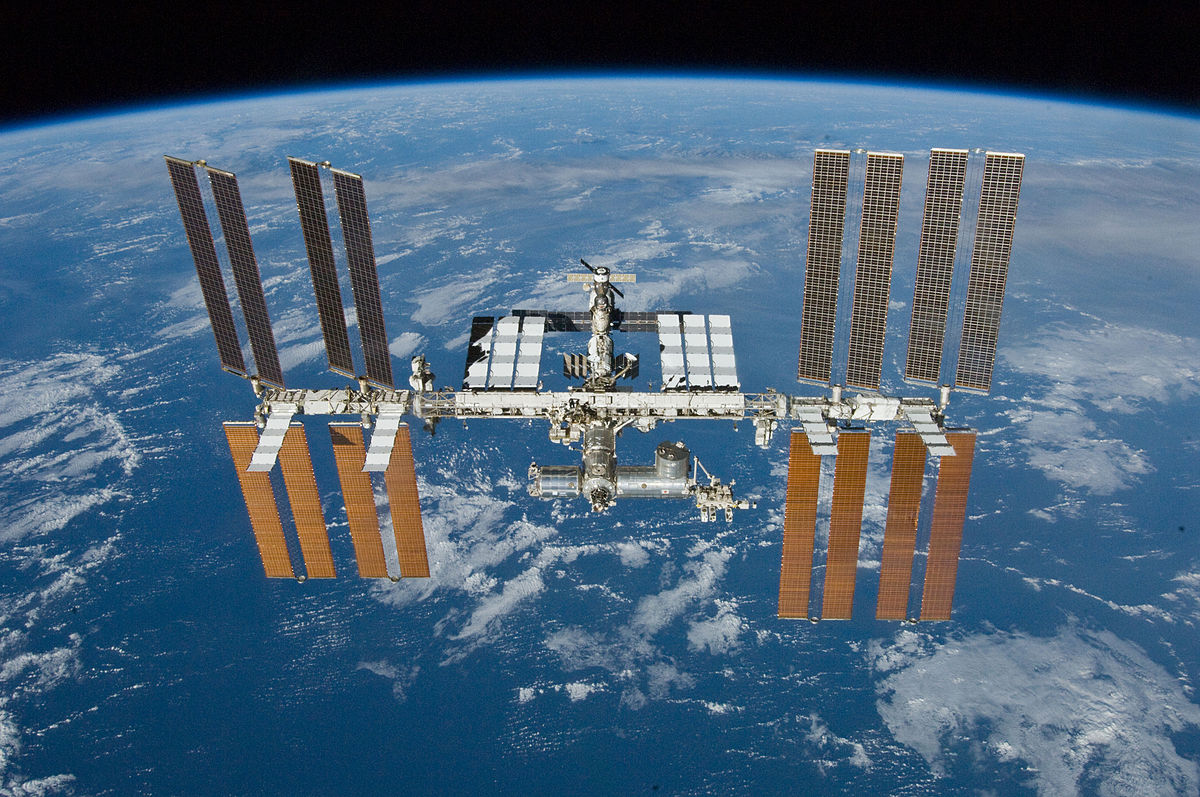
Living in space changes gene expression in humans
24.03.2017 / Scienceandmore / Category: Human Biology
NASA performed the first study of its kind with two identical twins, where one twin, Scott Kelly, lived on the International Space Station (ISS) for a full year, while his twin brother, Mark Kelly, stayed on Earth. Both identical twins were subjects to a wide range of test and investigations before, during and after the mission. The aim of this study was to gain further insight into the effects of extended time in space on the human body. It was found that many aspects of human physiology are impacted, which is not surprising per se. Staying in space, after all, is a tremendous amount of stress and the body needs to adapt.
However, some of the results are immensely interesting. The preliminary analysis of the collected data found that telomers are profoundly affected by staying in space. Telomers are the endings of the chromosomes and their function is to protect the coding DNA of the chromosomes from truncation during cell replication. This truncation of the DNA is an intrinsic characterisation of cell replication and happens with every replication. Telomers do not contain coding DNA and telomer shortening does not affect cell functions in general. However, when telomers become too short the cell often enters programmed cell death. Research suggests that shortened telomers are linked to age-related ailments and diseases (1). Scott Kelly’s stay in space significantly increased his telomer length, albeit it decreased to approximately pre-flight length within 48 hours of landing on earth. Telomer lengthening would essentially suggest a beneficial impact on health but it is hard to speculate whether this is actually the case, especially since the lengthening reversed so quickly after Scott Kelly’s return to Earth.
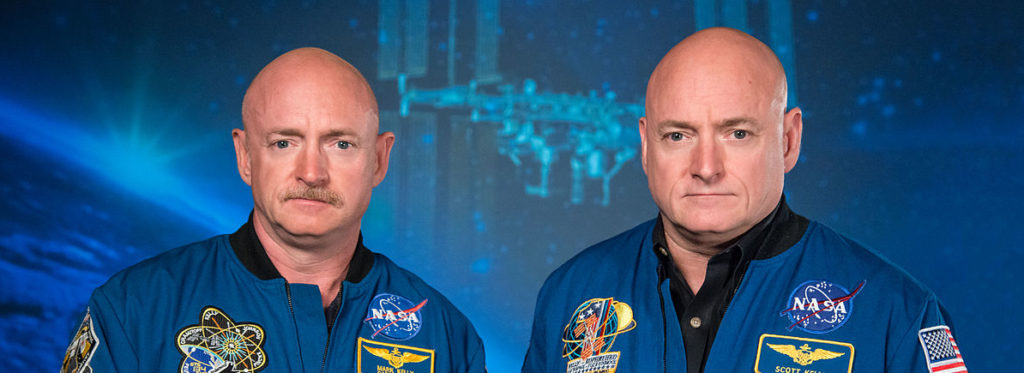
Scott Kelly (right) together with his brother, former astronaut, Mark Kelly (left).
Investigation on gene expression also indicated that Scott Kelly experienced higher levels of stress during his stay in space. However, it is somewhat unclear which DNA exactly has been investigated. One article states that the DNA and RNA of white blood cells has been used (https://www.nasa.gov/feature/how-stressful-will-a-trip-to-mars-be-on-the-human-body-we-now-have-a-peek-into-what-the-nasa) and another states cell-free DNA (https://www.nasa.gov/feature/nasa-twins-study-investigators-to-release-integrated-paper-in-2018) – cell free DNA contains DNA from white blood cells but also from other cells. Eventually, we need to wait for the publication of the actual research. Nevertheless, some genes were found to be differentially expressed only after spaceflight and were therefor labelled “space genes”. The alterations infer changes in several biological pathways in the cells. The impacted pathways are associated with: 1) tissue oxygenation and increased levels of carbon dioxide in the blood, 2) increased mitochondrial stress and increased occurrence of mitochondria in the blood, which indicates some form of damage to the mitochondria, 3) DNA damage repair, potentially caused by higher levels of radiation but also by Scott Kelly’s caloric restriction during his time in space, 4) bone formation, due to zero gravity, and 5) a hyperactive immune system, potentially caused by the different environment at the International Space Station. The majority of these changes in gene expression returned to post-flight levels but, surprisingly, 7 % of the astronaut’s gene expression remained disrupted 6 months after his return to Earth. This suggest possible longer-term changes induced by staying in space for one year.
Epigenetic investigations found an altered methylation pattern in several regions of the DNA of Scott’s white blood cells while he was on the ISS. These alterations returned to pre-flight state upon Scott’s return to Earth. This again could indicate potential genome damage that occurred in space. However, the results still need to be analysed.
Cytokine levels in Scott’s blood, which is a marker for inflammation, indicated increased inflammation. One set of cytokines was increased throughout his stay in space and another set of cytokines was increased right after his return to Earth. The latter even remained increased for six months. Additionally, certain proteins that are involved in maintaining normal insulin activity after meals were found to be increased. However, no speculations on the consequences have been made.
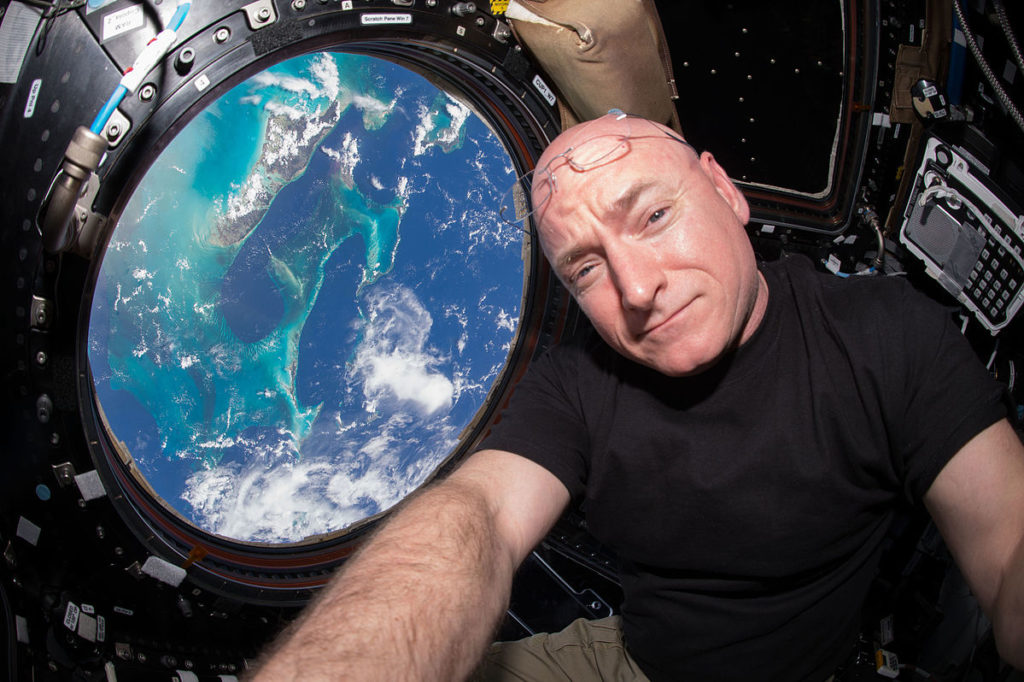
On the other side, staying in space for one year did not affect cognition, shown by several different tests. Neither was the immune systems response to a flu vaccine affected. The immune response of both twins to the flu vaccination was comparable and both showed increased levels immune cell production. This seems somewhat contrary to the gene expression investigations that suggested a hyperactive immune system.
Although, the gut microbiome differed pre-flight and during Scott’s stay in space, notably the bacterial taxa Bacteroidetes, one of the dominant phyla of the human gut microbiome (2), was decreased, the alterations did not persist upon his return to Erath. Overall, the changes were attributed to the change in diet during the stay at the ISS.
Cognitive investigations found that Scott Kelly’s speed and accuracy were decreased post-flight. This could, however, be attributed to exposure and adaption to Erath’s gravity.
This study aimed to gain further insight into the effects of extended time in space on the human body and contribute to the discovery of methods and technologies to support the safety of astronauts and their performance before, during and after space travel and exploration.
The preliminary publication of the results is vague, however draw interest and attention to the actual publication that is planned to be released by NSA 2018. So, stay tuned for further blog post on this exceptionally interesting study.
References
1. Eisenberg, D.T. (2011) An evolutionary review of human telomere biology: the thrifty telomere hypothesis and notes on potential adaptive paternal effects. Am J Hum Biol. 23(2):149-67.
2. Johnson, E. L., Heaver, S. L., Walters, W. A., & Ley, R. E. (2017) Microbiome and metabolic disease: revisiting the bacterial phylum Bacteroidetes. Journal of Molecular Medicine, 95(1): 1-8.