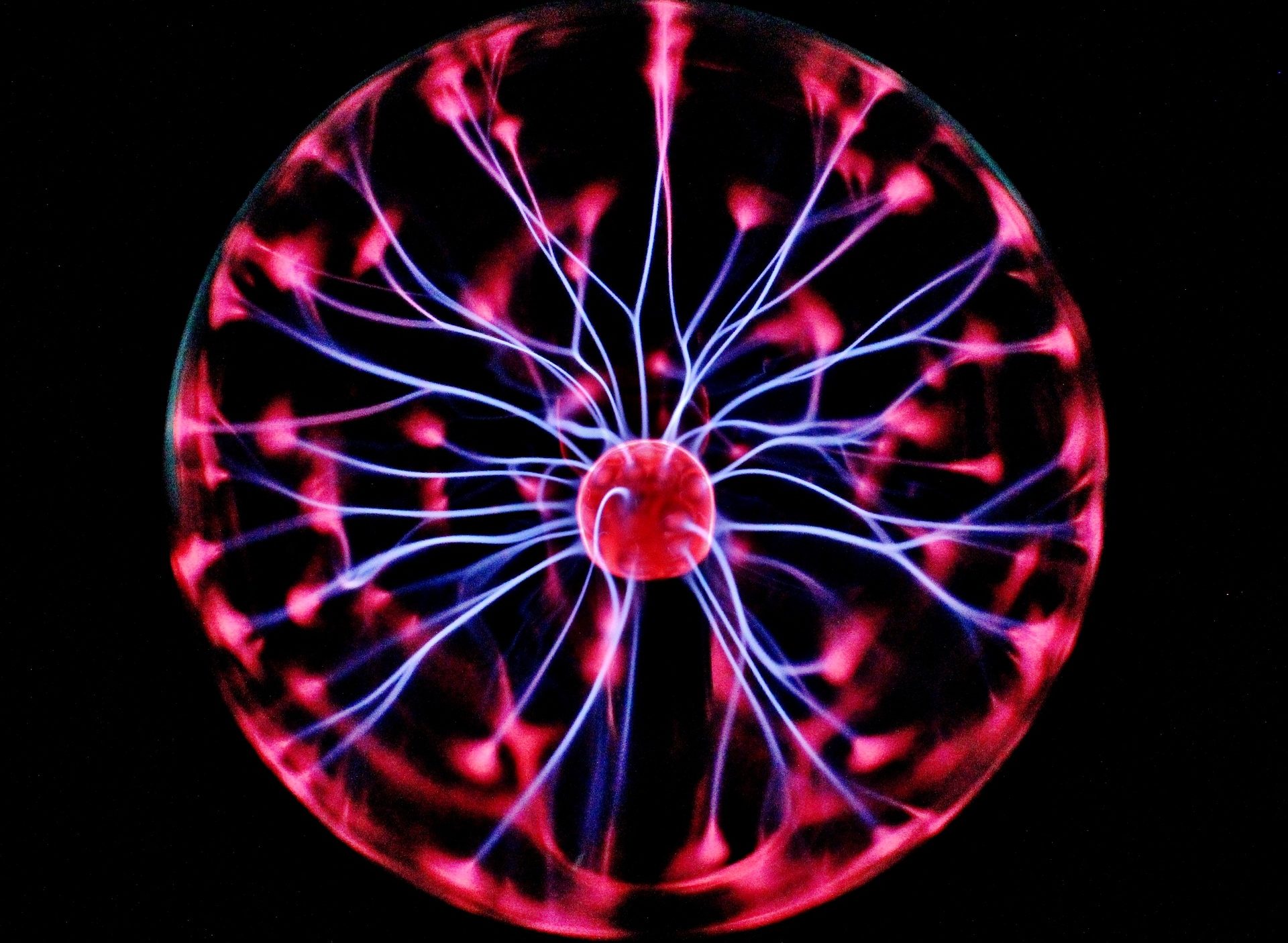
Cold plasma in seed, fruit and vegetable decontamination and plant disease control – Part 1
01.09.2017 / Scienceandmore / Category: Plant Biology
In the year 2050 the world’s population is estimated to have reached 9.7 billion, and worldwide crop production would have to increase two-fold in order to cover the demand by this time (United Nations, 2015; Tilman et al., 2011). An effective way to increase crop yield is minimising the losses to pathogenic microorganisms and insects by improving disease resistance of crop plants. Generally, this is done by selective breeding and application of disease treatments (fungicides, bactericides, insecticides). These approaches, however, could be accompanied by detrimental side effects. For instance, a constitutively activated plant defence responses could lead to an allocation of plant resources to defence response mechanisms instead of developmental processes and, in the worst case, cause decreased plant growth and yield (Heil et al., 2000; Heidel et al., 2004; Denancé et al., 2013; Huot et al., 2014). Plant pathogens could also become resistant to disease treatments which overall are deemed cost ineffective. Disease treatments additionally pose risks to the environment and consumers in long-term, especially due to the combination of different disease treatment residues in food products (Jørgensen et al., 2017).
A recently emerging alternative to conventional methods of improving plant disease resistance is cold plasma in the form of cold gas and cold solution plasma. Plasma describes matter which partly consists of charged components, ions and electrons, and is considered the fourth state of matter (besides, solid, fluid and gas) (Bourke et al., 2017).
Cold gas plasma is produced by subjecting atmospheric gases, such as air as gas mixture, to high energy via discharges (Bormashenko, et al., 2012, Jiang et al., 2014; Ziuzina et al., 2014) or microwaves (Kim and Min, 2017) at atmospheric pressure. This ionises the gas and generates reactive components like reactive oxygen species (ROS – they react very easily with molecules, which makes them potentially dangerous for living cells) and reactive nitrogen species (RNS – also very reactive), as well as UV-light, ions and electrons (Laroussi and Leipold, 2004; Laroussi, 2009; Heinlin et al., 2010; Oehmigen et al., 2010; Jiang et al., 2014; Panngom et al., 2014), but also nitrous oxide (N2O) and carbon dioxide (CO2), depending on the utilised gases (Ziuzina et al., 2014; Sivachandiran and Khacef, 2017). Since the temperature of gas plasma stays around room temperature during its generation, it is called cold gas plasma (Goossens, 2003). In nature, gas plasma is found in large quantities in earth’s ionosphere (Engwall et al., 2009).
Cold solution plasma or liquid-phase plasma is generated by exposing solutions (hexane and cyclohexane solutions for example) to high energy via microwaves, and by channelling air-based cold plasma through the solution, at atmospheric pressure. Plasma activated water (PAW) has also been generated by discharge (Takai, 2008; Bruggeman and Leys, 2009; Sivachandiran and Khacef, 2017). The temperature of solution plasma also remains at room temperature (Takai, 2008). In nature, PAW is generated during lightning that plasma-activates rain, and when lightning strikes water (Leenders, 2015).
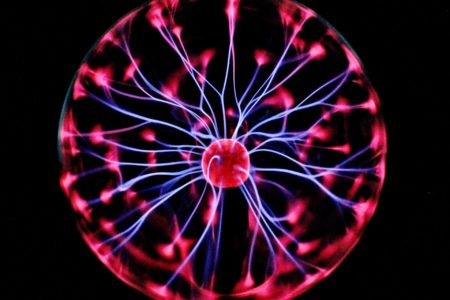
Significantly, investigations that were conducted thus far suggest that cold plasma mediates plant disease control, and improves seed germination and plant growth. Cold plasma has also been used for seed sterilisation in recent years (Volin et al., 2000; Takai, 2008; Sera et al., 2010; Leenders, 2015; Jiang et al., 2014; Ziuzina et al., 2014; Sivachandiran and Khacef, 2017).
Effect of cold plasma on microorganisms
The characteristic of cold plasma to generate reactive species, UV-light, ions and electrons infers the potential to inactivate microorganisms. For this purpose, application of cold plasma has been tested for microbial decontamination of solutions and disease control on plants.
Cold plasma and inactivation of microorganisms
Takai (2008) showed that cold sodium acetate (CH3COONa)- and sodium sulphate (Na2SO4)-based solution plasma killed E. coli (some strains can cause urinary tract infection in humans (Toval et al., 2014)) and Staphylococcus aureus (that can cause bacteremia in humans (Tong et al., 2015)) in highly concentrated suspensions after 30 seconds of treatment. Likewise, E. coli and S. aureus cell suspensions in water were inactivated within 5 and 15 minutes of continuous application of a nitric oxide (NO)-based plasma (Oehmigen et al., 2010). Plasma activated water, however, inactivated only some spores of the fungus Fusarium fujikuroi (a fungal pathogen of rice that causes Bakanae disease (Carter et al., 2008)) after exposure of 10 minutes (Kang et al., 2015). Cold argon plasma, on the other hand, effectively killed Fusarium oxysporum spores (that can cause wilt on several crop plants (Fravel, 2003; Di Pietro et al., 2003)) after 10 minutes of exposure (Panngom et al., 2014).
These results suggest that cold plasma, especially gas plasma, could be utilised to effectively decontaminate solutions such as wastewater (El-Sayed et al., 2015), but also that certain cold plasmas could be more suited for the inactivation of specific microbes in solution/water.
Cold plasma and seed decontamination
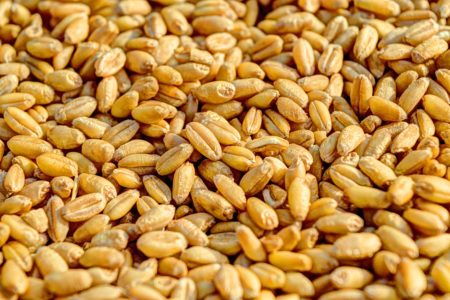
Seeds can harbour plant pathogenic microbes that are partially situated in the seed coat or in biofilms on the seed surface (Tsedaley, 2015; Danhorn and Fuqua, 2007) and can cause disease on seedlings after gaining sufficient quorum (minimum number to cause disease). Seed-borne pathogens are difficult to inactivate due to their location and, therefore, protection from outside influences. Seeds are commonly treated with different inactivating agents such as insecticides and fungicides, but also with heat and radiation (Wang et al., 2012; Sharma et al., 2015).
Investigations on the effects of cold plasma on crop seeds indicate a plasma mediated inactivation of microbes. Cold argon and air plasma treatments of rice seeds inactivated seed-borne pathogens and resulted in minor fungal infection of the emerging seedlings 14 days after germination. This was contrary to untreated seeds, where the emerging seedlings became heavily infected (Khamsen et al., 2016).
Plasma activated water was found to kill the spores on 80 % of F. fujikuroi infected rice seed. A scanning electron microscope analysis showed that almost all F. fujikuroi spores were detached from these seeds, contrary to control seeds, where fungal spores were still attached. This suggests that plasma additionally mediates mechanical detachment of spores from seeds (Kang et al., 2015).
Overall, cold plasmas were found to affect seed-borne pathogenic fungi and partly inactivate them. It could be speculated that due to the internal localisation of some microbes (and formation of biofilms), they were protected from cold plasma and survived the treatment, subsequently infecting the emerging seedling.
Cold plasma and inactivation of harmful microorganisms on fruits and vegetables
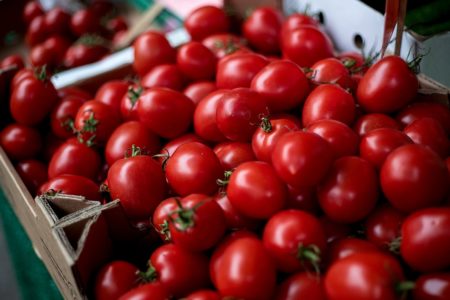
Vegetables and fruits can be a potential carrier of human pathogens. Food-borne pathogens have been found on lettuce, basil, sprouted seed, melon, tomatoes and radish (Rangel et al., 2005; Raybaudi-Massilia et al., 2009; Lim et al., 2010; Olaimat and Holley, 2012).
Cold plasma could be an alternative to commonly used agents such as organic acids and radiation to decontaminate fruits and vegetables. E. coli, Salmonella enterica (that can cause salmonellosis in humans (Andino and Hanning, 2015)) and Listeria monocytogenes (that can cause listeriosis in humans (Ramaswamy et al., 2007)) were effectively inactivated by cold air plasma on cherry tomatoes and apples (Ziuzina et al., 2014; Niemira and Sites, 2008). On strawberries that have a more uneven surface, however, cold air plasma treatment was less effective on these bacteria, but still reduced their concentrations (Ziuzina et al., 2014). Similarly, a different effectiveness of cold hydrogen peroxide (H2O2) solution plasma in inactivation of the E. coli strain O157:H7 (that can cause hemolytic uremic syndrome in humans (Lim et al., 2010; Rangel, et al., 2005)), Salmonella thyphimurium (that can cause gastroenteritis (Stephen et al., 1993)) and Listeria innocua (that can cause bacteremia in humans (Perrin et al., 2003)) was found on tomatoes, baby spinach leaves, tomato stem scars and cantaloupe rinds. H2O2 itself, without plasma activation, is utilised as antimicrobial agent but its effectiveness is considered as low (Ölmez and Kretzschmar, 2009). On tomatoes and spinach leaves, S. thyphimurium and L. innocua were inactivated much more effectively than on tomato stem scars and cantaloupe rinds. E. coli O157:H7 was only effectively inactivated by cold plasma on tomatoes (Jiang et al., 2017). These results suggest that rather uneven surfaces like the ones of strawberries could contribute to the survival of some bacteria (Ziuzina et al., 2014).
Overall, cold gas plasma shows potential in decontamination of food-borne microbes.
For this blog post, I used a lot of references. Please find a complete list at the end of Part 2